Key Takeaways
- Stochastic effects at pitches below 50 nm require a reevaluation of the practical resolution limit for EUV lithography, with significant observations at 24 nm half-pitch.
- LELE multipatterning technique, despite requiring multiple exposures, does not sufficiently mitigate stochastic effects due to the small critical dimensions printed.
- Comparative performance analysis indicates a slight improvement at 60 nm pitch relative to 30 nm pitch, but issues with stochastic defect rates persist.
Previously, I had indicated how detrimental stochastic effects at pitches below 50 nm should lead to reconsidering the practical resolution limit for EUV lithography [1]. This is no exaggeration, as stochastic effects have been observed for 24 nm half-pitch several years ago [2,3]. This then leads to the question of whether using multipatterning to get below the practical resolution limit can be of any help in avoiding these stochastic effects.
Multi-patterning in its most basic form involves forming a layer pattern with at least two mask exposures. In the simplest case, the LELE (Litho-Etch-Litho-Etch) approach, the target layer pattern is divided into two portions, which are combined by interleaving features such as lines. This is necessary when a single exposure cannot resolve (without defects, deformation, or feature loss) the minimum pitch between two features. The two features separated by this minimum pitch must then be exposed separately. For example, two 15 nm lines separated by 30 nm pitch would need to have two exposures: a first exposure to pattern one 15 nm line in resist, followed by an etch, and a second exposure to pattern the second 15 nm line in a subsequently recoated resist, followed by the etch.
Given that 15 nm lines on 30 nm pitch are known to be impacted by stochastic effects, it is natural to ask whether the same lines fare better on 60 nm pitch. Figure 1 shows a qualitative comparison. Without the actual mask structure details being known, a classical binary grating is used to represent the mask, and the illumination is the dipole used for 30 nm pitch, which will generated two beams from the mask for the 30 nm pitch case, and three beams from the mask (1st order being the middle beam) for the 60 nm pitch case. The same simulation model and conditions were used as in [1].
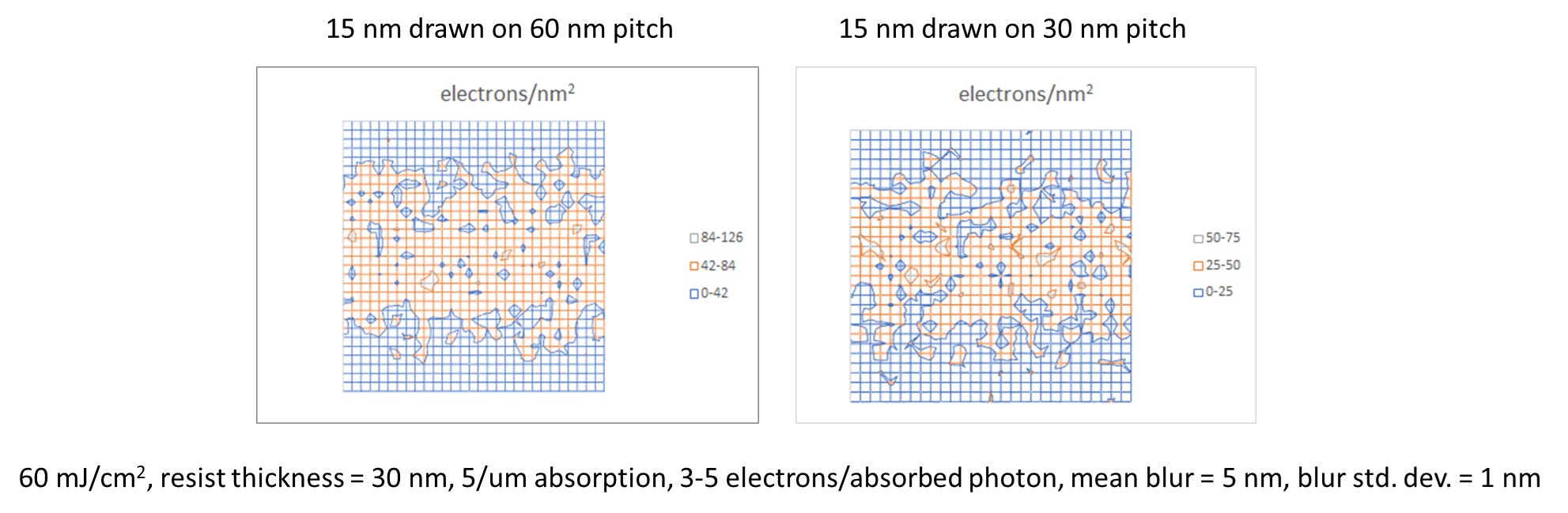
Figure 1. A 15 nm drawn line on 60 nm pitch (left) and 30 nm pitch (right). The same illumination is used for both cases (dipole for 30 nm pitch). The lines were modeled using binary grating features as the mask pattern. Under the shown modeling conditions, the 60 nm pitch is slightly better due to the higher photon and electron density in the exposed area.
The photon density happens to be higher for the 15 nm drawn line on 60 nm pitch. However, the NILS of the 15 nm line on 60 nm pitch is lower than on 30 nm pitch (1.7 vs. 2.8 even without blur). This means more pixels within the exposed region have a higher chance of the local photon density dropping below the threshold to become defective. The dark defect pixel % is a little lower for the 60 nm pitch compared to 30 nm pitch (~23% compared to ~30%). While it visually looks a little better, the edge definition from the scattered electron density is still poor. In addition, the stochastic defect rate has been found to be higher when the CD is less than the half-pitch [5,6]. Therefore, we must conclude that LELE patterning does not help avoid detrimental stochastic effects. The key reason is the CD being printed is still too small.
A way around this is to use spacers to define the CD. This is used in self-aligned double patterning (SADP) as well as SALELE (self-aligned LELE) [6]. This allows larger features to be printed by the exposure, e.g., 30 nm instead of 15 nm on 60 nm pitch. Interestingly, at around 40 nm pitch, double patterning by SADP or SALELE may overlap for DUV and EUV, since stochastic effects still look severe at 40 nm pitch [1], while 80 nm pitch is achievable by DUV single exposure [7].
Thanks for reading Exposing EUV! Subscribe for free to receive new posts and support my work. Pledge your support here.
References
[1] F. Chen, Stochastic Effects Blur the Resolution Limit of EUV Lithography.
[2] D. van den Heuvel et al., “Process Windown Discovery Methodology Development for Advanced Lithography,” ASMC 2016.
[3] S. Das et al, “E-beam inspection of single exposure EUV direct print of M2 layer of N10 node test vehicle,” Proc. SPIE 10959, 109590H (2019).
[4] P. de Bisschop and E. Hendrickx, “On the dependencies of the stochastic patterrning- failure cliffs in EUVL lithography,” Proc. SPIE 11323, 113230J (2020).
[5] J. Church et al., “Fundamental characterization of stochastic variation for improved single-expose extreme ultraviolet patterning at aggressive pitch,” J. Micro/Nanolith. MEMS MOEMS 19, 034001 (2020).
[6] F. Chen, SALELE Double Patterning for 7nm and 5nm Nodes.
[7] H. Hu et al., “K=0.266 immersion lithography patterning and its challenge for NAND FLASH,” CSTIC 2015.
Also Read:
Stochastic Pupil Fill in EUV Lithography
Application-Specific Lithography: Patterning 5nm 5.5-Track Metal by DUV
Why NA is Not Relevant to Resolution in EUV Lithography
Share this post via:
TSMC 2025 Technical Symposium Briefing